Scientific classification of the chernobyl “black fungus”
The black fungus found in Chernobyl’s radioactive ruins was identified as Cladosporium sphaerospermum, a dark-pigmented mold species. Its scientific classification is as follows:
- Domain: Eukaryota – (organisms with complex cells)
- Kingdom: Fungi – (the fungi kingdom)
- Phylum: Ascomycota – (sac fungi, characterized by spore-producing structures)
- Class: Dothideomycetes – (a class of mostly plant-associated fungi)
- Order: Capnodiales – (an order that includes sooty molds)
- Family: Davidiellaceae – (a family within the Capnodiales)
- Genus: Cladosporium – (a genus of common molds, often dark-colored)
- Species: Cladosporium sphaerospermum – the specific black fungus species thriving at Chernobyl (Cladosporium sphaerospermum – Wikipedia).
This fungus is one of several radiotrophic fungi discovered at Chernobyl, meaning it can grow in high radiation environments and potentially use radiation as an energy source (Cladosporium sphaerospermum – Wikipedia). Other fungi identified with similar traits include Wangiella (Exophiala) dermatitidis and Cryptococcus neoformans, all of which contain the pigment melanin in their cell walls.
Biological characteristics and unique properties
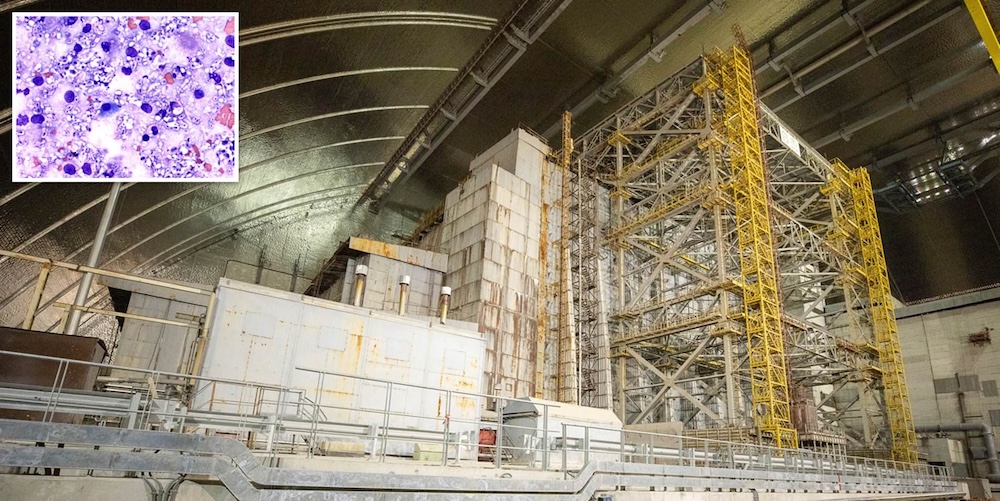
Cladosporium sphaerospermum is a dematiaceous fungus – it has darkly pigmented (black or olive-brown) cells due to high melanin content (Cladosporium sphaerospermum – Wikipedia). Some of its notable biological characteristics include:
- Morphology: It grows as a mold with branching septate hyphae (filaments) that are thick-walled and olivaceous-brown. Its colonies are usually velvety in texture and tend to remain flat rather than fluffy. The fungus reproduces mostly asexually by producing conidia (spores) in chains. The conidia are almost spherical (globose to ellipsoid, ~3–4 µm in diameter) and form in branched chains. This species can produce special larger conidia called ramoconidia at branching points, which help differentiate it from close relatives.
- Growth conditions: Interestingly, C. sphaerospermum is psychrophilic (cold-loving). It can grow at temperatures as low as -5 °C and up to about 35 °C, with optimal growth around room temperature (25 °C). It is also xerotolerant, meaning it tolerates low water availability or high salt conditions. These traits help it survive harsh environments.
- Radiotolerance: Its most unique property is its ability to withstand and even thrive in intense ionizing radiation. In the extremely radioactive environment of the Chernobyl reactor interior, this fungus not only survived but appeared to grow robustly and even orient its growth toward the radiation source. This unusual behavior is called “radiotropism,” meaning the fungus directs its growth toward radiation, similar to how plants grow toward light. Laboratory tests confirmed that many Chernobyl fungi grew towards strong beta and gamma radiation sources, suggesting the radiation itself was acting as an attractant or stimulus for growth.
- Melanin pigmentation: The dark color comes from melanin, a pigment in the cell walls. Melanin is known to protect organisms from ultraviolet radiation, but in these fungi it is thought to play an even more active role in capturing high-energy radiation. The heavy melanization is believed to be a key factor in the fungus’s radiotolerance and is implicated in its ability to harness radiation for growth.
In summary, the Chernobyl black fungus is a hardy, melanin-rich mold with extreme radiation resistance and the bizarre habit of “eating” or moving toward radioactive material. These properties set it apart as an unusual extremophile in the fungal world.
Mechanism of radiation absorption and conversion
How can a fungus “feed” on gamma radiation? The answer appears to lie in melanin, the black pigment. Researchers propose that these fungi perform a process dubbed “radiosynthesis,” analogous to photosynthesis, but using ionizing radiation instead of sunlight. While the exact biochemical pathways are still being unraveled, several studies have shed light on the mechanism:
- role of melanin: Melanin molecules can absorb a wide spectrum of electromagnetic radiation due to their electronic structure. In fungi like C. sphaerospermum, melanin likely absorbs gamma rays and helps convert that energy into a form the fungus can use. Experiments at the Albert Einstein College of Medicine showed that exposing melanized fungal cells (Cladosporium sphaerospermum, Wangiella dermatitidis, Cryptococcus neoformans) to high levels of radiation (500× background) led to increased growth and biomass accumulation compared to non-irradiated cells. Within 20-40 minutes of exposure, the fungi’s melanin showed altered chemistry and significantly enhanced its electron-transfer capabilities. In particular, irradiated melanin was able to reduce an electron acceptor (ferricyanide) using NADH about 3-4 times faster than melanin from unexposed cells. This implies the melanin was capturing radiation energy and funneling electrons into metabolic processes.
- radiosynthesis vs. photonsynthesis: Unlike photosynthesis (which involves multiple complex steps to convert light into chemical energy), the fungi’s process might be simpler. The term “radiosynthesis” is used to describe using ionizing radiation to drive metabolism. It’s hypothesized that melanin absorbs radiation and triggers electronic excitations or redox reactions that provide energy or growth benefits to the cell. For example, one study found that melanin exposed to radiation showed a four-fold increase in its capacity to reduce NADH, indicating energy transfer potential. In essence, melanin might function like a “radiant energy transducer,” capturing gamma photons and generating electrons or other chemical energy carriers that the fungus can use. This is still a subject of research, and scientists have not fully confirmed if the fungus can fix carbon (like plants do) using this energy or if it primarily helps the fungus more efficiently utilize other nutrients.
- radioprotection vs. energy harvesting: It’s important to note that melanin also provides protection. Melanin can physically shield cells and neutralize harmful free radicals produced by radiation. In highly radioactive environments, melanin-rich fungi suffer less DNA damage. The pigment is thought to quench reactive oxygen species generated by radiolysis (radiation breaking apart water molecules). So, melanin serves a dual purpose: it protects the fungus from radiation’s harmful effects and possibly channels some of that radiation energy into the fungus’s metabolism. Fungi engineered to be albino (no melanin) did not show enhanced growth under radiation, highlighting melanin’s key role.
In summary, the black fungus’s melanin acts like a “radio-reactive battery.” It soaks up gamma rays, undergoes changes in electronic structure, and boosts metabolic processes (like electron transfer and growth) in the fungus. This remarkable mechanism allows the fungus to convert deadly radiation into usable energy – a phenomenon at the frontier of our understanding, with researchers still working to fully decode the biochemistry behind this form of energy conversion.
Discovery in chernobyl and scientific studies
Early observations in the chernobyl ruins
Not long after the 1986 Chernobyl nuclear disaster, scientists exploring the wreckage made a startling observation: the highly radioactive interior of Reactor 4 was turning black with fungal growth. In 1991, five years post-accident, researchers reported that the walls of the ruined reactor were covered in a black mold-like fungus. Even in the cooling pools of water, fungi were growing and turning the water black with their pigmented spores.
Ukrainian mycologist Nelli Zhdanova and colleagues were among the first to study these fungi. They found “hot particles” of reactor graphite (highly radioactive debris) that were being actively colonized and broken down by the fungi. The fungi didn’t just happen to live there – they appeared to prefer the radioactive material. Zhdanova’s team coined the term “radiotropism” to describe how the fungal hyphae grew directionally toward sources of beta and gamma radiation. In controlled experiments, about two-thirds of fungal isolates (many from Chernobyl) grew toward a radiation source in Petri dishes, while genetically similar fungi from non-radioactive areas showed little or no such attraction. This ruled out mere coincidence and suggested a true biological phenomenon of radiation-seeking behavior.
Over the next 15 years, thousands of strains of hundreds of microfungal species were isolated from the Chernobyl exclusion zone. Many were darkly pigmented species (rich in melanin) such as Cladosporium, Alternaria, Penicillium, and yeasts like Cryptococcus. The most frequently encountered was Cladosporium sphaerospermum, which became emblematic of Chernobyl’s “black fungi”. Remarkably, some of these fungi could even decompose the radioactive graphite moderator blocks from the reactor core – literally “eating” the reactor’s remains, likely using both the carbon source and the radiation energy.
Breakthrough laboratory studies
Initial findings raised a provocative question: were these fungi actually growing better because of the radiation, not just in spite of it? To investigate, Dr. Ekaterina Dadachova and Arturo Casadevall led pioneering studies in the mid-2000s. In 2007, they published a seminal paper demonstrating that certain Chernobyl fungi grew faster in the presence of ionizing radiation than without it. They grew melanin-rich fungi (Wangiella, Cryptococcus, etc.) under high gamma radiation in the lab and observed significant increases in biomass and acetate uptake compared to control conditions. The fungi’s metabolism and gene expression were altered by radiation exposure, indicating a real physiological response to harness the energy. This was the first direct evidence supporting the idea of radiosynthesis.
Dadachova’s 2007 study also delved into the chemistry: after radiation exposure, fungal melanin showed changes consistent with electron excitement (detected via electron spin resonance) and became more efficient at energy transfer (reducing NADH). In essence, it was as if the melanin were “charged up” by the radiation, analogous to how chlorophyll is energized by sunlight. They concluded that melanin could indeed act in energy capture and utilization for fungi, opening the door to considering radiation as a possible nutrient or fuel source for life.
Multiple studies since then have reinforced these findings. A 2008 review by Dadachova & Casadevall summed up that C. sphaerospermum was one of the predominant species in the reactor and that melanized fungal cells exhibit enhanced growth after irradiation. In 2004, Zhdanova’s group formally published evidence of radiotropism in Mycological Research, and other researchers examined genetic changes in fungi from Chernobyl that might underlie their extreme radiation resistance. More recently, modern genomic and transcriptomic studies (Malo et al. 2021) have looked at how fungi like Exophiala dermatitidis adapt at the molecular level when exposed to radiation, finding shifts in stress response genes and melanin production pathways.
In 2020, an intriguing study from the University of Saskatchewan showed that fungi can even be “trained” to better sense and move toward radiation. By exposing black fungi to radiation over time (radio-adaptation), they induced a heightened growth response when the fungi later encountered high-dose radiation. This suggests we may enhance or select for fungi with super-efficient radiosynthesis capabilities.
Why chernobyl?
The Chernobyl reactor provided a unique environment – high radiation, abundant decaying organic material (like dead trees, structural materials, and graphite), and little competition from other organisms (most plants and animals died or left). These conditions likely selected for fungi that could survive the radiation and exploit it. By 1991, scientists noticed the soil and surfaces “turning black” from fungal growth in contaminated zones. It’s a striking example of life adapting to what we would consider an uninhabitable environment. Chernobyl’s black fungus has since become a cornerstone example of extremophiles, organisms thriving under extreme conditions – in this case, extreme radioactivity.
Potential applications of radiation-loving fungi
The discovery that fungi can “eat” radiation and possibly convert it into useful energy has spurred a wave of innovative ideas. From space travel to environmental cleanup and medicine, radiotrophic fungi and their melanin pigment have promising applications. Here are some of the exciting possibilities:
1. Space travel and radiation shielding
One of the biggest challenges in long-term space travel (such as a mission to Mars) is exposure to cosmic radiation. Astronauts outside Earth’s protective magnetic field face radiation levels far higher than on Earth, which can damage tissues and electronics. The Chernobyl fungus offers a potential solution: living radiation shields.
Researchers, including NASA scientists, have experimented with C. sphaerospermum aboard the International Space Station (ISS). In a 2019-2020 experiment, a sample of this fungus was grown on the ISS to test its ability to block space radiation. The results were promising: even a thin layer of fungus measurably reduced radiation levels. In fact, a 1.7 mm thick layer of C. sphaerospermum on a Petri dish led to about a 2.4% reduction in ionizing radiation compared to a control, demonstrating roughly five times greater radiation shielding than an equal mass of non-living material. While 2-3% reduction is small, it’s significant for such a thin biofilm. The experiment showed that the more fungal biomass, the more radiation was attenuated.
Scientists projected that if you surrounded an object (or person) with the fungus, the shielding could cut radiation by ~4%. To reduce radiation on the Martian surface to Earth levels, calculations suggest you’d need about a 21 cm thick layer of this fungus as a shield. That may sound thick, but because the fungus can grow and self-replicate, astronauts wouldn’t need to lift all that shielding material from Earth – they could potentially grow it on-site (a concept of self-regenerating shields). Mixing fungal melanin with Martian soil could also improve shielding while keeping weight down; one concept is a composite of regolith (Martian soil), melanin, and fungal biomass about 9 cm thick to achieve substantial protection.
Beyond blocking radiation, the idea of using the fungus as a space “power plant” is being pondered. If the fungus indeed converts radiation to chemical energy, astronauts might harness that metabolic activity. For instance, some have speculated about energy storage systems where radiotrophic microbes supplement solar energy, almost like living solar panels that use cosmic rays. While this is still speculative, the fungus could at least act as a protective coating on habitats, suits, or spacecraft, and even repair itself if damaged by radiation. Because it’s a living system, it can heal and continue growing, offering an advantage over static shields.
Importantly, melanin itself (without the live fungus) is being tested as a radiation-protective material. In 2019, a team from Johns Hopkins sent purified fungal melanin to the ISS to examine how well it protects against cosmic rays. If melanin proves effective, we might see melanin-based paints or films shielding space equipment and perhaps lining spacecraft walls in the future.
2. Bioremediation of nuclear waste and environments
Another exciting application is using these fungi to clean up radioactive pollution on Earth. Since Chernobyl, scientists have realized these fungi can absorb radioactive isotopes and lock them into their cells. The melanin-rich cell walls can bind heavy metals and radionuclides, effectively removing them from the environment.
Potential uses in bioremediation include:
- Cleaning contaminated soil: In areas like the Chernobyl Exclusion Zone or Fukushima, radio-adapted fungi could be introduced to contaminated soil to soak up cesium, strontium, and other dangerous isotopes. The fungi would “bioaccumulate” these elements. Because they also tolerate high radiation, they would survive where plants or bacteria might die off. After the fungi have grown, they can be harvested, and the radioactive material is concentrated in the fungal biomass, which can then be safely contained or disposed of. This process is a form of mycoremediation (fungus-based remediation). Fungi have already been shown to immobilize radionuclides in Chernobyl’s soil. Notably, they even consumed some of the organic radioactive debris (like the graphite) in the reactor, reducing its mass.
- Containing nuclear accidents: Ekaterina Dadachova’s team suggests “training fungi” to detect and accumulate radioactivity. These trained fungi could serve as biosensors – for instance, spread over an area where a dirty bomb or an illicit nuclear test is suspected; the fungi would grow more vigorously near radiation hotspots, effectively flagging those areas. As they grow, they’d also encapsulate radioactive particles, preventing them from leaching into groundwater or spreading. This concept could aid in monitoring and cleaning sites of nuclear accidents or decommissioned reactors.
- Waste treatment: Fungi might also be used in nuclear waste management facilities. They could form a layer in waste repositories to catch any leaking radionuclides. Some yeasts and fungi are extremely radiation-resistant and can also tolerate toxic heavy metals. Research on fungal species in radioactive waste has found they often outperform even bacteria in survival and sequestration of radionuclides.
One challenge is what to do with the radioactive fungi after cleanup. The biomass itself becomes nuclear waste. However, since the fungi concentrate the radioisotopes, they greatly reduce the volume of contaminated material, which is a win for waste management. The concentrated fungal biomass can be incinerated in controlled conditions (ash can be stored) or possibly processed to extract useful isotopes.
3. Medicine and radioprotection for humans
The melanin from these fungi could inspire new ways to protect humans from radiation or even treat certain conditions:
- Radiation shielding for patients and workers: Just as melanin shields fungi, it might shield human cells. Scientists have proposed creating a sort of “sunblock” against radiation using fungal melanin. For example, cancer patients receiving radiation therapy could potentially take melanin or have it applied to protect healthy tissues during treatment. Nuclear power plant workers or airline pilots (who get extra cosmic ray exposure) might use melanin-based supplements or creams to reduce DNA damage. In fact, experiments have shown that mice given fungal melanin or even diets of certain melanin-rich mushrooms had better survival rates after lethal radiation doses. In one study, mice that received melanin from a fungus (Gliocephalotrichum simplex) had higher survival, healthier spleens, and less oxidative damage after being irradiated, compared to control mice. The melanin seemed to help by boosting cell signaling pathways (like restoring an important protein, ERK, that radiation knocks down).
- Drugs and therapeutics: There is interest in developing radioprotective drugs from melanin or mimicking its structure. Melanin is a natural antioxidant and radical scavenger ( Ionizing Radiation: how fungi cope, adapt, and exploit with the help of melanin – PMC ) ( Ionizing Radiation: how fungi cope, adapt, and exploit with the help of melanin – PMC ). A melanin-based compound could be given to astronauts or first responders in nuclear emergencies to prevent radiation sickness. Since melanin is a biopolymer, one challenge is delivering it (it’s insoluble). Some approaches include melanin nanoparticles or injectable forms. Notably, melanin from fungi is chemically similar to the pigment in certain edible mushrooms, so even dietary avenues are being explored (though one would have to eat a lot of mushrooms to have an effect!).
- Medical imaging and cancer treatment: Interestingly, melanin’s ability to absorb radiation could be useful in targeted cancer therapy. Scientists have considered whether melanin-producing microbes could be used to concentrate radiation in a tumor (though this is far-fetched and in early imagination stage). More practically, melanin’s radioprotective trait could shield healthy cells during cancer radiotherapy, as mentioned above.
- Biosensors for health: The radiation-sensing ability of the fungus might even be used in small detectors for monitoring radiation exposure. Imagine a personal dosimeter that has a tiny vial of melanized fungus – if radiation levels go up, the fungus’s metabolism changes, which could trigger a readable signal. This is speculative but demonstrates how biology might assist technology.
4. Biotechnology and other technologies
Beyond space and medicine, radiotrophic fungi and their pigments have other innovative uses:
- Energy harvesting: The concept of harvesting energy from radiation is intriguing. Nuclear batteries exist (like RTGs – radioisotope thermoelectric generators), but those use decay heat. Fungi offer a biological approach. If we could engineer a system where fungal melanin converts gamma rays to electrical energy (for example, via electron transfer reactions), we might create a kind of biological radiation battery. Researchers have noted that melanin pigments can produce an electrical current in certain conditions, and there’s ongoing research in bioelectrochemistry looking at melanin from microbes to see if it can be used in fuel cells or sensors.
- Sensors and detectors: As mentioned, trained fungi could act as living sensors. They might be used to detect illegal nuclear material. Also, melanin’s conductivity changes when it’s irradiated (as Dadachova showed). This means a melanin-coated circuit could potentially serve as a real-time radiation detector, changing its electrical resistance or current when radiation is absorbed.
- Industrial biotech: Fungal melanin is extremely stable and can bind various substances. It’s being explored for use in filtration (to remove toxins or even nerve agents, since melanin can bind chemical pollutants). The porosity and binding capacity of melanin could make it a component in filters or protective gear for chemical/radiological defense.
- Materials science: The idea of incorporating melanin into materials (plastics, paints, fabrics) for radiation shielding is attractive because melanin is lightweight and biocompatible. For instance, melanin-infused polymers might coat the interior of airplanes or space stations to reduce chronic low-dose radiation. Even consumer electronics could benefit (to protect sensitive components from background radiation or to harden devices for high-altitude flight).
- Energy storage: One speculative notion mentioned by researchers is using the fungi for energy storage. Since they convert radiation to chemical energy, perhaps they could charge some kind of biological capacitor or store energy in chemical form that can be tapped later. This is far-out biotechnology that would require significant advances, but it’s on the table as a long-term idea.
From protecting astronauts to cleaning nukes, the applications of the Chernobyl fungus span many fields. It’s a prime example of how an odd discovery in a disaster zone can inspire cross-disciplinary innovation.
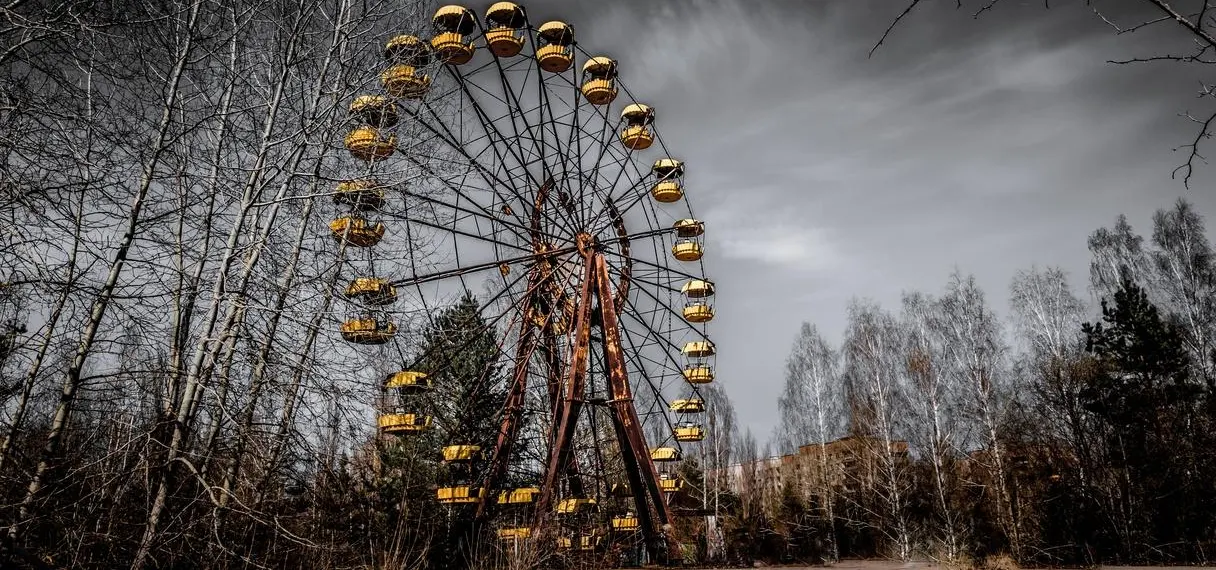
Ethical and safety considerations
While the potential is exciting, using a radiation-eating fungus in practical applications raises important ethical and safety questions:
- Pathogenicity and health risks: Many melanin-producing fungi are opportunistic pathogens or allergens. Cladosporium sphaerospermum itself is generally considered a common environmental mold and can cause allergies or asthma in sensitive individuals. Other related “black molds” and Cryptococcus neoformans (one of the radiotrophic fungi) can cause serious infections in people with weakened immune systems. Working with or deploying such fungi in the environment or in space habitats must ensure they don’t pose a risk to human health. If we were to coat a spacecraft or a room with live fungus, we’d need to be sure it wouldn’t inadvertently infect the crew or produce toxic spores. Scientists are exploring using just the melanin pigment (which is not infectious) as an safer alternative to live fungal cultures for applications involving human exposure ( Fungal Melanins and Applications in Healthcare, Bioremediation and Industry – PMC ) ( Fungal Melanins and Applications in Healthcare, Bioremediation and Industry – PMC ).
- Containment and control: Introducing a hardy fungus to a new environment (like Mars, or a lab, or a nuclear site) requires careful control. These fungi can spread via microscopic spores. There’s an ecological ethics aspect: if we release radiation-hungry fungi in a contaminated site, could they unintentionally spread beyond the target zone? We wouldn’t want an invasive species scenario. However, in places like Chernobyl’s reactor or a sealed waste facility, this is less of a concern since the area is already hostile to most life and contained. In space, any biological material needs quarantine protocols to prevent contaminating other planets (planetary protection rules) – ironically, a fungus that thrives on radiation might survive interplanetary travel better than most microbes, so we must be cautious.
- Genetic modification: There may be interest in genetically enhancing these fungi for better performance (e.g., a GMO fungus that produces extra melanin or grows faster). Any GMO used outside would raise regulatory and ethical questions. For instance, could modified fungi transfer genes to native species? Could they evolve in unexpected ways in the wild or in space? Thorough risk assessments would be needed.
- Post-cleanup disposal: Using fungi for bioremediation leads to the issue mentioned: the fungi become radioactive. Handling and disposing of radioactive biomass must follow nuclear safety protocols. There’s an ethical component in ensuring that workers involved in such bioremediation are not put at undue risk and that the waste is secured. Some might argue the process simply moves contamination from one form to another, but the counterargument is that it concentrates and contains it, which is still beneficial.
- Unknown ecosystem effects: Fungi that absorb radiation could alter the microecosystems where they are applied. For example, in soil cleanup, as fungi dominate, they might outcompete other microbes or change soil chemistry. We’d need to monitor such effects to avoid harming soil health long-term. However, in already devastated zones like Chernobyl’s reactor core, fostering fungal growth might actually be rehabilitative since not much else lives there.
- Ethical use of extremophiles: There’s a broader philosophical question: do we have the right to exploit extremophile life forms for our benefit without fully understanding their ecology? Some ethicists suggest caution in meddling with organisms that have very specialized niches. That said, these fungi are naturally occurring and already in these environments, and using them to mitigate human-caused disasters (like nuclear spills) might be seen as a positive ethical duty to heal the environment.
In all cases, the safety protocols will likely favor using isolated melanin or dead fungal biomass rather than actively growing cultures when possible. For instance, making melanin-based coatings avoids the risk of live fungus. If live fungi are used (like in space or cleanup), they would likely be kept in closed systems or barriers to prevent uncontrolled spread. As research continues, scientists are mindful of these concerns and often highlight the need for more knowledge about fungal melanin and handling of melanotic fungi ( Fungal Melanins and Applications in Healthcare, Bioremediation and Industry – PMC ) ( Fungal Melanins and Applications in Healthcare, Bioremediation and Industry – PMC ).
Recent research and future prospects
Research into Chernobyl’s black fungus and its applications is rapidly advancing, bridging microbiology, biophysics, and engineering. Here are some recent findings and future directions:
- International space station experiments (2018–2022): The fungus C. sphaerospermum has now been tested in space. The preliminary tests in 2018-19 on the ISS (by NASA and collaborators) showed that the fungus could grow in microgravity and offer radiation shielding as discussed. A follow-up study published in 2022 reported that the fungus not only grew well on the ISS but actually had a slightly higher growth rate in orbit compared to on Earth, possibly due to the constant radiation exposure (~1.2× growth increase). This hints at a radioadaptive response to space radiation where the fungus might be benefiting from the cosmic rays. Additionally, the experiment confirmed a detectable reduction in radiation underneath the fungal culture relative to a no-fungus control, attributable to the fungal biomass absorbing radiation. These findings pave the way for scaling up the idea of living radiation shields in space. Future ISS experiments are likely to test thicker fungal layers and longer durations, and even co-culture with Martian soil simulants to see how a hybrid shield might work.
- Molecular insights (2020–2021): Scientists have been probing the genomes and gene expression of radiotrophic fungi. A 2021 study by Malo et al. looked at how the gene activity of Exophiala dermatitidis (a radiotrophic black yeast) changes after radiation exposure. They found activation of stress response pathways and upregulation of genes related to melanin production and DNA repair under high radiation. Similarly, a 2022 study (Bland et al., in Scientific Reports) investigated how exposure to gamma rays and UV affected the growth and pigmentation of related fungi. Interestingly, they observed significant increases in melanin pigment production after radiation, even if growth rate didn’t always increase. This supports the idea that fungi boost melanin (for protection and possibly energy capture) when living in radioactive environments.
- Radiation-sensing fungi (2020): As noted with the University of Saskatchewan work, researchers have managed to enhance fungi’s radiation response by pre-exposing them (essentially training them). This adaptive training could lead to fungal strains optimized for particular isotopes or radiation levels. It’s akin to selective breeding for the best “radiation-sniffing” fungus. Future research may produce custom fungi for specific cleanup tasks – for example, a strain particularly good at accumulating cesium-137 from soil, or one that forms a thick biofilm shield quickly when radiation is present.
- Melanin engineering: There’s growing interest in synthesizing or modifying melanin for use outside the fungus. Melanin can be extracted from fungal cultures in large quantities (it’s a hardy polymer). Teams are exploring composite materials with melanin, like mixing fungal melanin into plastics or fabrics to create radiation-protective gear. In 2021, an in-depth review on fungal melanins concluded that while the potential is high, challenges like melanin’s complex structure and isolating it efficiently need to be overcome ( Fungal Melanins and Applications in Healthcare, Bioremediation and Industry – PMC ) ( Fungal Melanins and Applications in Healthcare, Bioremediation and Industry – PMC ). Future materials science breakthroughs might allow tailoring melanin’s properties (for example, making it more electrically conductive or more flexible) to integrate into devices.
- Expanding to other extremes: Radiotrophic fungi have also been found in other extreme places – for instance, on the International Space Station’s exterior and in high-altitude environments. Some were even exposed on the outside of the ISS (a project called EXPOSE-E) for a year and survived cosmic rays and vacuum. These species, like Cryomyces antarcticus, maintained high survival and low mutation rates, credited to their melanin protection. This tells us that radiotrophic fungi (or at least their spores) could potentially endure interplanetary travel. Future astrobiology research might examine if such fungi could hypothetically survive on Mars’ surface or help seed life support systems in habitats there.
- Commercial and industrial research: Agencies like NASA and the European Space Agency (ESA) are actively looking at biotechnologies for radiation shielding, so funding and interest in these fungi is on the rise. Startups are even looking into melanin-based coatings (for electronics, satellites, etc.). Also, defense agencies are curious if melanin or fungal derivatives could protect soldiers from radiation or chemical exposure. In the energy sector, nuclear facilities are considering bio-monitoring methods (like fungal sensors) as part of safety systems. This cross-sector interest suggests a vibrant future for bringing this science out of the lab.
- Open questions: Despite progress, several questions remain for the future. Scientists are still trying to conclusively prove how much of the fungus’s growth energy can come directly from radiation. It could be that radiation augments their metabolism rather than completely substituting for food. As one review put it, it’s not yet shown if fungi can fix carbon (turn CO₂ into biomass) using radiation energy alone. Future experiments may involve culturing the fungi in minimal media with radiation to see if they can sustain themselves with very little other nutrients. Another open question is whether we can transfer this ability to other organisms – could we, for instance, engineer a plant or bacterium to use melanin and gain radiotrophic capabilities? Such synthetic biology work might be far off, but it’s conceivable.
In conclusion, the humble black fungus from Chernobyl has gone from a scientific curiosity to a promising tool for innovation. Ongoing research is likely to unlock more of its secrets, making what was once science fiction (organisms feeding on radiation) into practical reality. From protecting future Mars explorers to cleaning our planet’s worst nuclear messes, this radiation-loving fungus exemplifies how life can adapt in astonishing ways – and how those adaptations can be harnessed for the betterment of technology and society.
References:
- Zhdanova, N. N. et al. (2004). Ionizing radiation attracts soil fungi. Mycological Research, 108(9), 1089–1096.
- Dadachova, E. et al. (2007). Ionizing radiation changes the electronic properties of melanin and enhances the growth of melanised fungi. PLoS One, 2(5): e457.
- Dadachova, E. & Casadevall, A. (2008). Ionizing Radiation: how fungi cope, adapt, and exploit with the help of melanin. Curr. Opin. Microbiol., 11(6), 525–531.
- Shunk, G. K. et al. (2020). A Self-Replicating Radiation-Shield for Human Deep-Space Exploration: Radiotrophic Fungi on the ISS (preprint).
- Cordero, R. J. et al. (2021). Fungal melanins and applications in healthcare, bioremediation and industry. Microorganisms, 9(7): 1465 ( Fungal Melanins and Applications in Healthcare, Bioremediation and Industry – PMC ) ( Fungal Melanins and Applications in Healthcare, Bioremediation and Industry – PMC ).
- Peachey, C. (2020). Chernobyl fungus used in space experiments. Nuclear Engineering International.
- University of Saskatchewan News (2020). USask researchers training fungi to sense radiation and clean up nuclear waste.
- Royal Society of Biology (2019). Eating gamma radiation for breakfast – The Biologist (Feature by Tom Ireland).
- Bland, J. et al. (2022). Evaluating changes in growth and pigmentation of fungi in response to gamma and UV irradiation. Sci Reports, 12:12142.
- [Additional citations inline above from TechnologyNetworks, Wikipedia (Cladosporium sphaerospermum – Wikipedia), etc., provide further reading and source data.]